Issue 5: Reality?
The Unseen Reality Visualizing Phenomena through Living Systems

The Unseen Reality Visualizing Phenomena through Living Systems
By Nancy Diniz and Frank Melendez of Augmented Architectures
A vision of biological systems that will augment our bodies, not just with data, but also with through enhanced senses that might produce an awareness of the ephemeral and the invisible.
Our perception of our surroundings is governed by our senses, particularly via signals through our skin. We use these messages to interpret extreme temperature, atmosphere, moisture, and the like. In comparison to the sensorial technology at our fingertips, our human skin’s ability to analyze the reality of our environment can seem limited. Technologies are converging to drastically change the way we look at the relationships in the world around us. Innovations like autonomous robotics, ubiquitous sensing networks, and cyber-physical and synthetic biological systems give artists and designers the opportunity to redefine human-machine-environment interactions. This research investigates the opportunities for the design of wearable devices to incorporate traditional electronic and bio-sensing, computational form-finding, and manufacturing processes to visualize biometric and environmental phenomena.
Many creatives, informed by a rich artistic and filmic lineage, use these technological advancements to explore the notion of augmentation, projection, or expansion of the human skin (Cruz and Spize, 2005). This practice is built upon a history ranging from the hybrid forms of 16th century Bruegel to the visceral aesthetics in David Cronenberg’s work, most vividly the flesh formations of the game-pods in the 1999 film eXistenZ. Stelarc, an inspiring visionary artist, presented different ways of altering the body in order to adjust and extend “its awareness to the world” (Teyssot 2005). This amplification thus calls for a remapping of the body with new designs and reconfigurations, considering the expansion of human skin as a living landscape without a true limit or contour; a symbiotic ecosystem between the body and the external environment.
Growth and Simulation of Living Organisms
Looking to the behavior of living organisms as inspiration, we explored the possibility of demonstrating this ecosystem by growing, harvesting, and manufacturing responsive biological layers. Let’s explore “Artificial Lucidum” and “Embryonic Spaces”, two projects featuring responsive devices that augment the physical form with biometric sensing capabilities. This ongoing research is predicated on interdisciplinary collaborations within the fields of design, computation, and biotechnology. These projects present a novel approach to architectural design research in machinic instruments that emerge as morphological responses to biotic and abiotic phenomena at the interface of bodies and spatial ecological systems. Through the use of biometric and environmental sensing, these small-scale wearable devices operate as liminal mechanisms, creating a dynamic boundary between the body and the environment. These technologies alter our ability to imagine constructed systems, requiring a nuanced and expanded view of networked and object-oriented relationships between internal bodily signals and surrounding atmospheric data. With this, inhabitants of buildings, for example, are not treated solely as users acting within a static environment, but as stakeholders with agency who act as catalysts for architecture that can adapt to changing materials, and environmental or ecological demands.
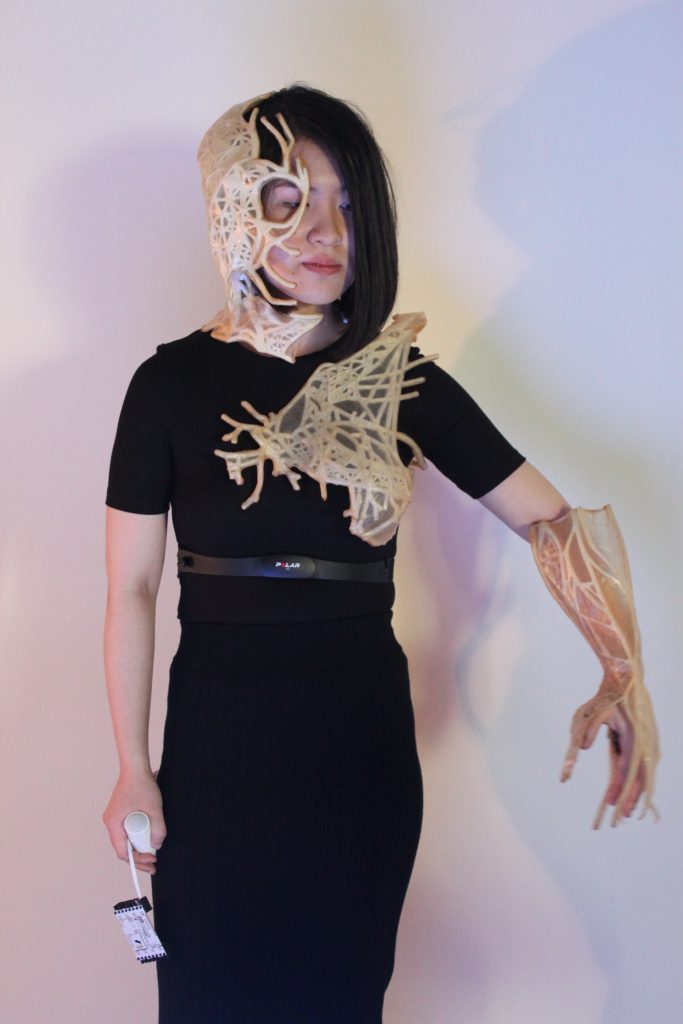
Figure 1. The post-human body augmented with three wearable architectural devices. Image credits ©Augmented Architectures
“Embryonic Spaces,” 2016
The design process starts by growing materials to create “body architectures” (Figure 1), developing a framework for architecture as an interface and extension of the human body. This is achieved through the implementation of biofabrication processes and sensing technologies that utilize and integrate internal body signals and atmospheric flows in determining the body-machine-environment relationship.
In the project entitled “Embryonic Spaces”, we used bacterial cellulose as a means of growing biomaterials for three wearable devices. Microbial cellulose has proven to be remarkably versatile and can be used in a wide variety of fields to produce items such as paper products, electronics, and biomedical devices. We experimented with the Acetobacter strains, in particular the Acetobacter Xylinum bacteria, which is the same strain used in Kombucha tea and the most common and efficient type for a series of material testing samples. The ingredients necessary for bio-fabricating the bacterial cellulose are water, tea, sugar, and vinegar. The cellulose is developed through the fermentation process of bacteria, glucose, and oxygen within the water. Nanofibers of cellulose are spun into layers by bacteria, forming a sheet on the surface of the water. The material sheets can be removed from this aquatic environment in three-week cycles and dried to produce a translucent sheet. Synthetic biological processes offer the potential to grow these materials into specific forms and shapes for the biofabrication of architecture. (Figure 2).
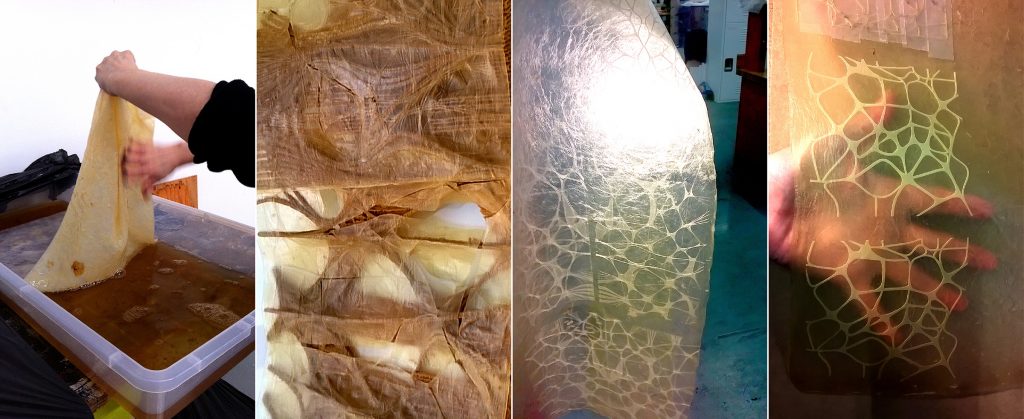
Mapping, Modeling, and Fabrication of the Body Devices
We have adapted a strategy of creating forms from the inside out, mapping internal processes that would materialize into second skins, connected with different biometrics sensing on each piece. We decided to couple the following body parts with biometric sensing technology – the arm piece (cardiovascular system with heart-rate sensors), the chest and back piece (organs and muscle anatomy with dermal activity) and the head piece (cardiovascular system and organs with brain activity).
Each piece senses bio-data in real time. The project integrates physical computing methodologies using code, microcontrollers, and sensors that measure biometric information, including heart rate, thermal activity, and brain activity. This includes the use of Python and Javascript; Arduino and BITalino microcontrollers; and electrocardiograph (EKG), electrodermal (EDA), and electroencephalography (EEG) sensors, respectively. Each of the three prototypes is embedded with hardware and focuses on a specific biometric by allowing users to connect the sensors to specific locations on their bodies (Figures 1 and 4). Biometric data is sensed and output through lighting, sounds, and digital patterns that visually and auditorily express this invisible, ephemeral phenomenon.
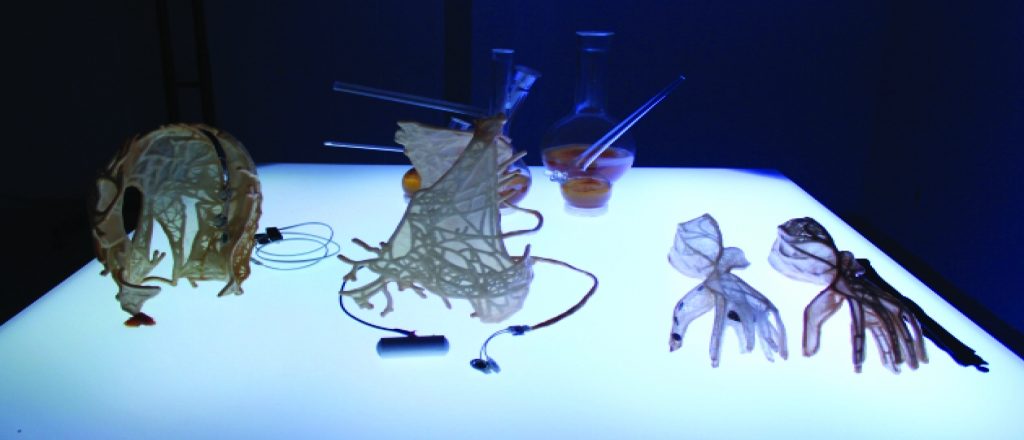
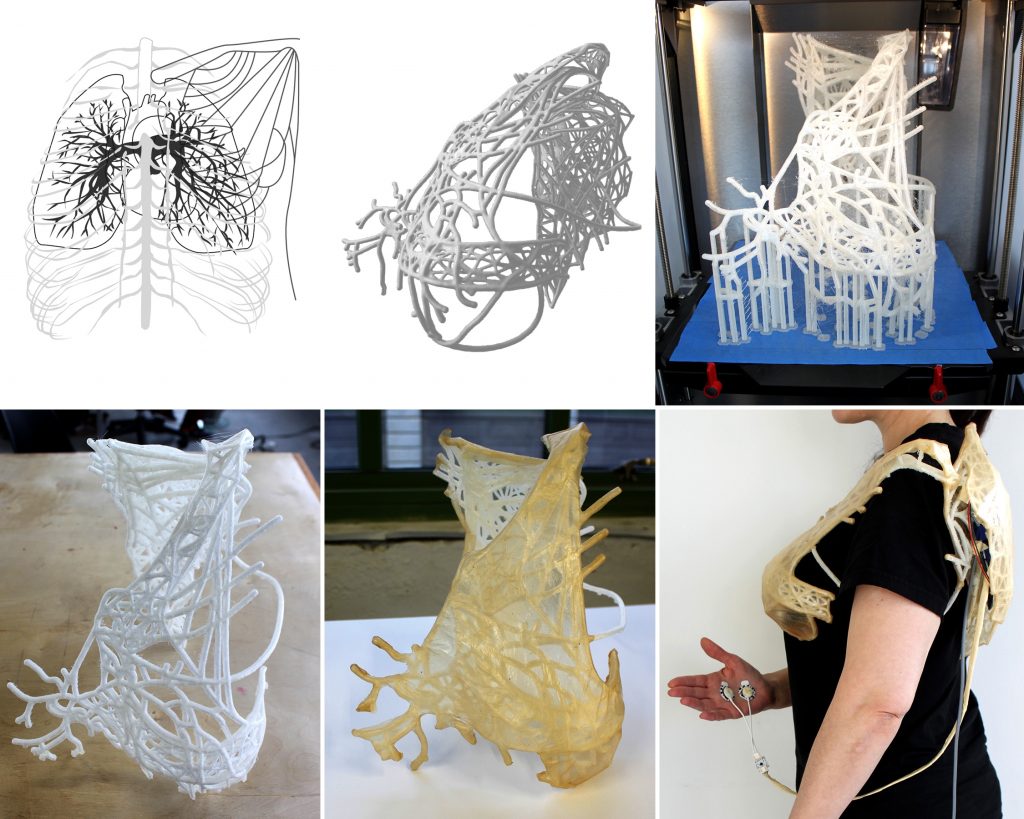
Figures 3 and 4. Design and fabrication process of the chest piece wearable device: anatomical diagram, digital model, 3D print on the MakerBot Replicator Z18 printer, final 3D print, bacterial cellulose clad prototype, and final prototype of wearable device with sensors and hardware integration. Image credits ©Augmented Architectures
“Artificial Lucidum,” 2018
These bespoke devices are intended to serve as wearable architectures that augment the body by providing the user with data pertaining to environmental toxins. There are a total of three devices, located on the wrist, the arm, and the shoulder. (Figures 1, 3 and 4). The form of the 3D-printed wearable devices contain various sinuous geometries, apertures, and pockets that allow for the placement of the E. coli bacteria biosensors. As environmental toxins in the atmosphere increase, the E. coli bacteria glows to indicate the levels of sulfur dioxide, UV-light levels, and heavy metals. This serves as a responsive architectural interface that enhances the user’s sense of reality.
In this project, a series of rules were established to set up a framework for a variety of growth experiments that explore the patterns and morphologies of E.coli and Physarum polycephalum. These living organisms were grown under specific conditions within various parameters, allowing for a set of criteria with which to assess their natural growth pattern and unruly behavior. “Artificial Lucidum”’ adapts a bio-inspired form-finding method using Physarum polycephalum, a single-celled organism commonly known as slime mold, that can aggregate to produce efficient, multi-cellular spatial structures. This behavior was then studied using agent-based computational design simulations to generate the structure of the architectural devices. Spaces within these devices are allocated to grow E.coli bacteria, which act as biosensors that detect the presence or absence of environmental toxins, such as ozone, UV-light levels, heavy metals, and sulfur dioxide. Due to their high sensitivity to surrounding environment and rapid response to external stimulants, cells like microalgae or E. coli bacteria can act as so-called bioreceptors.
Bacterial biosensors are created using DNA cloning technology, followed by bacterial transformation. DNA cloning produces an intended protein by expressing specific genes at high levels of E. coli. For example, genes coding for the green fluorescent protein (GFP) can be used as reporter genes, resulting in a bio-fluorescent effect. (Figures 5 and 6). The embedded, living E. coli bacteria have very specific nutrient needs, a rapid reproduction rate, and a very short life cycle, making them an ideal source of pollution assessment as part of a wearable biosensor. To express genes that produce reporter proteins, a full-length double-stranded cDNA (or copy DNA) is isolated. Next, the double-stranded cDNA is inserted into an expression vector, which is adapted to function in E. coli. To express the protein in E. coli, the cDNA must be transcribed from an E. coli promoter (usually T7, derived from a bacteriophage). A promoter is a region of DNA that initiates (or signals) the expression of the reporter gene.
In our project, we imagine that the binding of environmental toxins (small molecules like sulfur dioxide, sulfur, and metal ions) to the promoter regions will act as a signal to produce GFP, emitting a green light visible under UV light. We theorize the use of genetically modified bacteria, yielding different fluorescent colors according to the environmental toxin they are reacting to, resulting in green fluorescence in the presence of high UV-light levels, magenta in the presence of heavy metals, and cyan in the presence of sulfur dioxide. Each color of bacteria was tested in petri dishes filled with different 3D-printed patterns of agar (Figure 6). Using augmented reality, the environmental toxins are visualized as quantitative data through a mobile phone app interface. With this, users can understand the invisible aspects of the specified pollutants that are present in their surrounding environment.
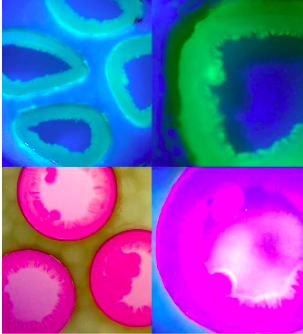
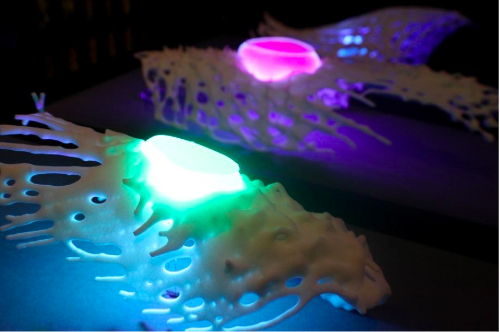
Figures 5 and 6. Physarum polycephalum growth experiments. ©Augmented Architectures.
Towards a Semi-Living Materiality
Some of the current limitations to bacteria biosensors include the reliance on UV-light in order for the E. coli to fluoresce. Our next step in the research will be to genetically modify the bacteria to bio-illuminate, which will eliminate the need for external light sources to visualize data and changes in the bacteria. Additionally, genetically modifying the E. coli bacteria to produce particular odors (like wintergreen or banana) can introduce an olfactory approach to perceiving changes in the environmental condition. The project aims to engineer biological systems that respond to external phenomena, but also serve as informative, intelligent systems that provide users with data, allowing for devices that augment bodies through enhanced senses, producing a greater sense of environmental awareness.
References
- Armstrong, R. and Ferracina S. Ed (2013) Unconventional Computing: Design Methods for Adaptive Architecture, Designed and edited (with Rachel Armstrong). Published by Riverside Architectural Press.
- Araya, Sergio, Ekaterina Zolotovsky, and Manuel Gidekel (2012) Living Architecture: Micro Performances of Bio Fabrication, In Physical Digitality: Proceedings of the 30th eCAADe Conference, 447-457. Vol. 2. eCAADe: Conferences 2. Prague, Czech Republic: Czech Technical University in Prague, Faculty of Architecture, 2012.
- 2009 Cruz, Marcos; Pike, Steve (guest-eds.). AD – Neoplasmatic Design, Wiley – 136 pages ISBN: 978-0-470-51958-5.
- Gama, M., Gatenholm P, Klemm, D. Edited (2013) Bacterial NanoCellulose: A Sophisticated Multifunctional Material, CRC Press.
- Haraway, D. J. (1991) A Cyborg Manifesto: Science, Technology and Socialist-feminism in the late twentieth Century, in Simians, Cyborgs and Women – the reinvention of Nature, Routeledge New York 1991.
- Hayles, K (1999) How we Became Post-human: Virtual Bodies in Cybernatics, Literature, and Informatics (Chicago: University of Chicago Press, 1999)
- Leach, Neil. 2004. “Swarm Tectonics” In Digital Tectonics, edited N. Leach, D. Turnbull and C. Williams. New York: Princeton Architectural Press. 70-77.
- Ma, Yidong and Wieguo Xu, 2017. “Physarealm: A Bio-inpired Stigmergic Algorithm Tool for Form-Finding” In Protocols, Flows and Glitches, Proceedings of the 22nd International Conference of the Association for Computer-Aided Design Research in Asia (CAADRIA) 2017, edited by P. Janssen, P. Loh, A. Raonic, M. A.
Schnabel. Hong Kong: 499-509. - Teussot, G (2005) Hybrid Architecture: An Environment or the Prosthetic Body, SAGE Publications, London, Vol 11(4): 72-84.
- Newman, Timothy and Hong Yi, 2006. “A survey of the marching cubes algorithm” In Computers & Graphics 30.
- Sawer, Eric. “Bacterial Odor Generators” In Bio 2.0: Dissecting the next revolution in biology (Blog).
Augmented Architectures is a research and design practice developing projects that operate in-between the human body and architectural scale and engage topics pertaining to biotechnology, digital and bio fabrication, computational design, virtual reality, interactive design and data visualization. They curate international exhibitions, symposia and workshops and create interactive installations, wearable devices, sustainable material systems, and drawings as extended notions of architecture.
Nancy Diniz is a registered architect and educator practicing in New York City. Nancy is the Director of the Masters in Biodesign at Central Saint Martins, University of the Arts London. She is the recipient of numerous grants and fellowships namely from New York State Council on the Arts/Storefront, MacDowell Colony, EYEBEAM, Arctic Circle, US, Seoul Art Space Geumcheon, Korea and The Foundation for Science and Technology, Portugal. She is author of the upcoming book ‘Unstable Matters – Designing with Living Organisms’.
Frank Melendez is a New York based architect and educator. Frank is an Assistant Professor at the City College of New York, Bernard and Anne Spitzer School of Architecture. Frank is author of the book “Drawing from the Model; Fundamentals of Digital Drawing, 3D Modeling and Visual Programming in Architectural Design” and has published texts that focus on the role of responsive technologies in architectural design and educational pedagogies. These works have been supported through grants and fellowships, including the MacDowell Colony, in which he was awarded a 2017 Artist-In-Residence fellowship.